Application Note
Translation of NK Cell CAR Therapy to the Clinic: Critical Role of Performance & Clinical-scalability
Background
The prognosis for adult B-cell malignancies, such as acute lymphoblastic leukemia, non-Hodgkin lymphoma and chronic lymphocytic leukemia, is poor, particularly for patients with refractory or relapsing disease.1- 4 Recently, two breakthrough anti-CD19 CAR-T cell therapies were approved by the FDA for use in adults with specific relapsed or refractory B-cell malignancies.
These CAR-based cell therapies can lead to dramatic clinical responses and represent a substantial step forward in the treatment of B-cell malignancies. However, they face significant challenges for wide-spread adoption, the foremost of which is the cost and complexity of manufacturing. Significant drivers of the cost and complexity are the means of cell engineering and the autologous nature of the treatment.
NK cells represent a promising avenue for cell therapy as they: i) rapidly kill tumors in an antigen-independent fashion; ii) represent an allogeneic source of cells for simplified manufacturing and treatment of patients with compromised immune systems; and iii) have established clinical safety and modest anti-tumor efficacy upon transfer of autologous or allogeneic cells.
NK cells have historically proven difficult to engineer. First generation NK-CAR engineering using viral transduction demonstrated the cytotoxicity of anti-CD19 CAR NK cells against B-cell malignancies, however it posed challenges for more sophisticated engineering, commercial manufacturing and ultimately sacrificed clinical translation.
Non-viral engineering of NK cells using MaxCyte® electroporation technology provides significant improvements in efficiency and viability as well as a clinically-feasible, FDA-approved avenue to overcome manufacturing challenges and advance the field of CAR cell therapy.
Aim
Develop and validate MaxCyte's cell engineering technology as a safe, clinically feasible means of anti-CD19 CAR expression in primary NK cells. Specifically, demonstrate reproducible, large-scale manufacturing of anti-CD19 CAR NK cells using mRNA electroporation as well as establish in vitro functionality, in vivo safety, and anti-tumor activity
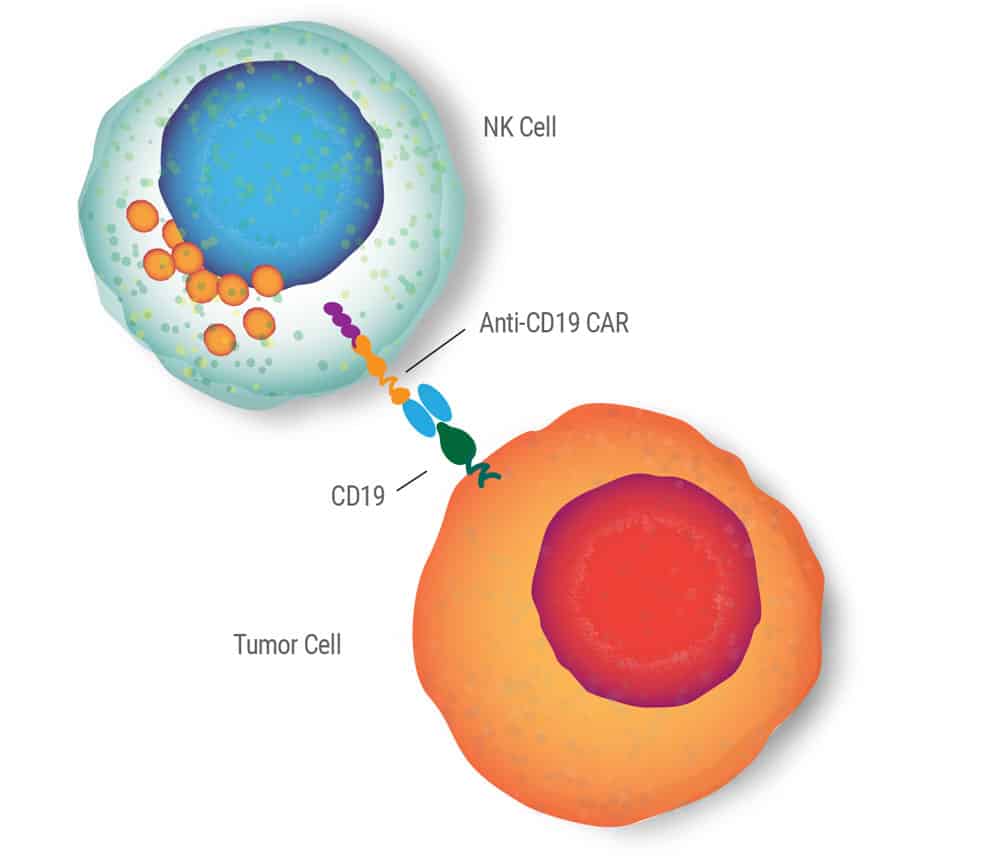
NK Cell Electroporation
- Peripheral blood mononuclear cells were collected and co-cultured with K562-mb15-41BBL cells for 7 days followed by T cell depletion resulting in ≥94% CD56+ CD3- cells.
- 1x107 to 1.5x107 NK cells were resuspended in 50 μL of MaxCyte Electroporation Buffer and mixed with 150-200 μg/mL of CD19-BB-ζ mRNA for small-scale electroporation on the MaxCyte GT. Up to 1x109 NK cells were electroporated using 100 μg/mL of CD19-BB-ζ mRNA for large-scale studies.
- Electroporation was performed using MaxCyte recommended protocol.
- Post electroporation, cells were resuspended in RPMI-1640 or SCGM media with 10% FBS and 100 IU/mL IL-2.
Full methods for in vitro assays and in vivo anti-tumor studies are detailed in Cytotherapy, 14(7), 830-840, 2012.
Results
> 80% Efficiency and > 85% Viability Using mRNA Electroporation
NK cells expanded from 9 healthy donors were electroporated with mRNA encoding the anti-CD19-BB-ζ receptor to evaluate baseline transfection efficiency, cell viability, and consistency using unoptimized conditions (i.e. mRNA concentration and NK cell density). 24 hours post electroporation 70% of NK cells (range 57-84%) expressed the anti-CD19 CAR with 90% (range NK Cells 81-95%) cell viability (Figure 1). Subsequent large-scale electroporation of NK cells from 12 individuals using optimized conditions resulted in 82% CAR expression and 87% cell viability.6
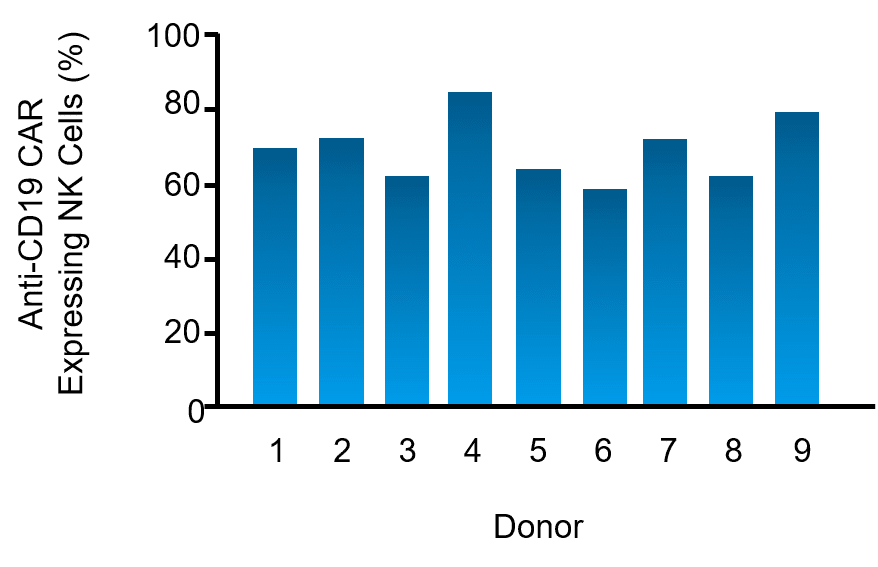
Figure 1. Expanded NK cells from nine healthy donors were electroporated with mRNA encoding an anti-CD19 CAR. CAR expression on NK cells was assessed via flow cytometry 24 hours post electroporation.
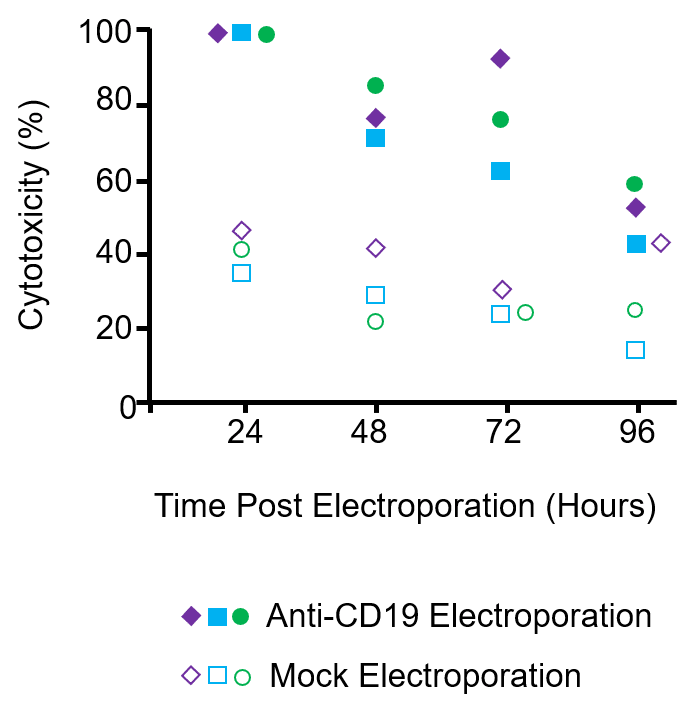
Figure 2. NK cells expanded from three donors were electroporated with anti-CD19 CAR mRNA or mock electroporated (no mRNA). Cytotoxicity against the CD19+ 380 cell line was assessed over the course of 4 days via a 4-hour cytotoxicity assay at a 1:1 effector-to-target ratio.
Significant Enhancement of In Vitro CD19-specific Effector Functions
In vitro functionality of the engineered NK cells was assessed 24 hours post electroporation including CD19-specific IFN-γ production and cytolytic activity. Co-culture of NK cells with the CD19+ 380 cell line resulted in over 2x the IFN-γ production compared to mock electroporated NK cells from the same donor.6
Engineered NK cells expressing the anti-CD19 CAR exhibited considerably higher cytotoxicity against CD19+ B cell lymphoma cell lines, including 380, OP-1, RS4; 11, Raji and Ramos, compared to mock electroporated cells. Importantly, CAR-expressing cells did not exhibit increased cytotoxicity against CD19– tumor cell lines or against non-transformed allogeneic mesenchymal cells.6 Kinetic analysis of cytolytic activity showed that increased CD19-specific cytotoxicity correlated with CAR expression, remaining statistically higher over the 4-day study (Figure 2).
10 Day Reduction in Manufacturing
Side-by-side experiments were conducted using expanded NK cells from three donors in which cells were either transduced with a retrovirus containing a CD19- BB-ζ construct using previously established protocols or electroporated with CD19- BB-ζ mRNA.7 At peak expression 60% (39, 59 & 80%) of transduced cells expressed the anti-CD19 CAR. In contrast, 87% (75, 91 & 94%) of electroporated cells from the same three donors expressed anti-CD19 CAR and at higher expression levels than transduced cells. No differences in CD19-specific cytotoxicity were detected between the two engineering methods on a per cell basis, however, the absolute number of NK cells expressing the CAR was substantially higher following electroporation.
Retroviral transduction required two consecutive days of infection followed by 8-10 days of culturing to reach peak CAR expression. Alternatively, mRNA electroporation was performed in less than 1 hour with peak CAR expression as early as 24 hours post electroporation, saving as much as 10 days in the manufacturing process. Additional manufacturing time and cost savings of MaxCyte mRNA electroporation result from the relative simplicity of mRNA preparation versus the manufacturing and testing of viral stocks as well as improved consistency.
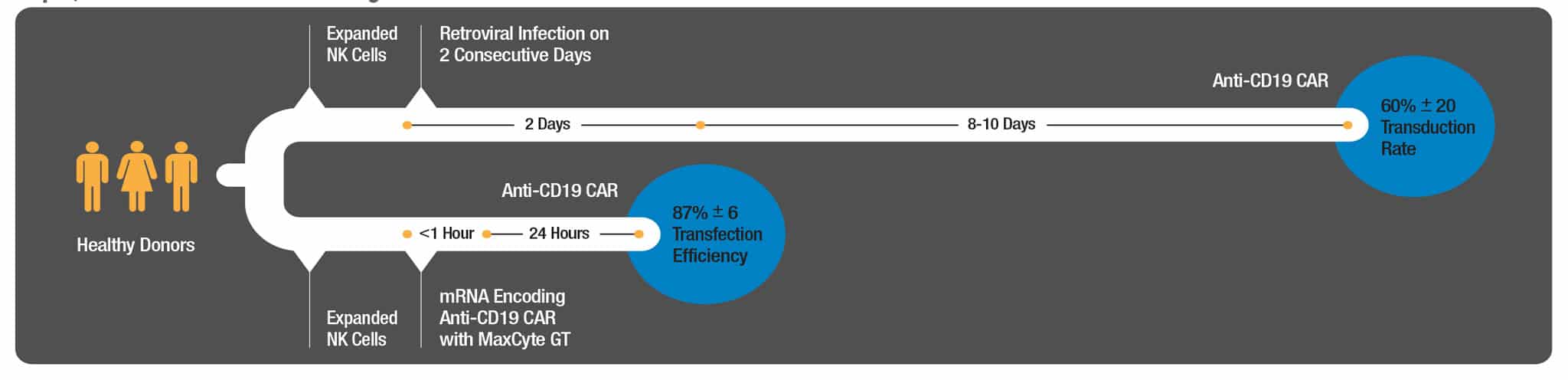
Figure 3. NK cells expanded from three donors were either electroporated with anti-CD19 CAR mRNA or subjected to retroviral transduction on two consecutive days with a MSCV containing the anti-CD19-BB-ζ construct. CAR expression, viability and cytotoxicity were assessed 24 hours post electroporation or 8-10 days post retroviral transduction.
10-fold Lower In Vivo Tumor Burden
Considerable in vivo cytotoxicity of anti-CD19 CAR NK cells was observed in a xenograft model of B-cell leukemia. NSG mice were injected either intraperitoneally (i.p.) or intravenously (i.v.) with a luciferase expressing CD19+ 380 cell line, followed by injection of CD19 CAR- or mock-electroporated NK cells.6 Leukemia progression was assessed using luciferase imaging. Mice that received NK-CAR cells either i.p. or i.v. showed markedly decreased (approximately 10-fold) tumor burdens over the course of the 30 day experiment.
Initiation of Human Clinical Trial
CAR expression was detectable as early as 6 hours post electroporation, peaked at 24-48 hours, and became statistically insignificant after 5 days.6 This induced strong NK cell in vitro effector functions, such as IFN-γ production and CD19- specific cytolytic activity, that were maintained for 4 days. In vivo xenograft models of B-cell leukemia using mRNA engineered, NK-CAR cells resulted in 10-fold lower tumor burdens demonstrating the ability of these cells to mediate in vivo anti-tumor activity. This suggests that NK cells engineered via MaxCyte mRNA electroporation will have a beneficial therapeutic effect despite the transient nature of CAR expression. These studies opened the path to a clinical trial (Clinical Trial #: NCT01914479) using large-scale MaxCyte GT-engineering NK cells.
Conclusion
These studies established the ability of MaxCyte’s regulatory-compliant electroporation platform to engineer primary NK cells at a clinical-scale with higher viability, efficiency, and consistency compared with established virus-based engineering methods. MaxCyte mRNA electroporation saved 10 days of manufacturing time, significantly lowered costs, and importantly resulted in enhanced NK cell engineering that translated into improved in vitro and in vivo anti-tumor efficacy. Based on MaxCyte’s solid foundation of performance, payload flexibility, and clinical-scalability, developers can perform sophisticated engineering and gene editing to augment the efficacy of current CAR therapies and rapidly advance novel NK cell therapies to clinic.
References
- Treating childhood acute lymphoblastic leukemia without cranial radiation. (2009) N Engl J Med 360: 2730-2741.
- Adult acute lymphoblastic leukemia: concepts and strategies. (2010) Cancer 115: 1165-1176.
- Ongoing improvement in outcomes for patients diagnosed as having non-Hodgkin lyphoma from the 1990s to the early 21st century. (2008) Arch Intern Med 168: 469-476.
- Update on therapy of chronic lymphocytic leukemia. (2011) J Clin Oncol 29:544-550.
- Expression of chimeric antigen receptors in natural killer cells with a regulatory-compliant non-viral method. (2009) Cancer Gene Ther 17(3):147-54.
- A clinically adaptable method to enhance the cytotoxicity of natural killer cells against B-cell malignancies. (2012) Cytotherapy, 14(7): 830-840.
- Genetic modification of primary natural killer cells overcomes inhibitory signals and induces specific killing of leukemic cells. (2005) Blood 106(1): 376-383.